BYPASS VALVES/BOV ARE NOT WASTE GATE RELIEF VALVES
Contrary to most perceptions a bypass valve is designed to relieve boost/pressure when the throttle blade is closed to idle or part throttle openings. Conditions such as idle, during shifts, and backing off throttle and light load driving conditions it is very important to have a bypass valve. A bypass valve utilizes a vacuum line port behind the throttle blade, and activates when detecting an immediate change in pressure. Bypass valves/BOVs are different from a Wastegate valve, which operates as a Boost pressure operated relief valve and opens to release a proportion of exhaust pressure when a certain Inlet pressure is achieved, this is associated with Turbos to control turbine speed and hence power to drive the Compressor.
With Supercharged engines during non-boost driving, bypass valves can reduce fuel consumption and NOX emissions and allow the Supercharger to operate at a cooler temperature and load by circulating compressed air (boost) back to the inlet, it also reduces the parasitic losses of driving the Supercharger to compress/move air when it is not needed. This is NOT a concept unique to only Eaton Superchargers. It is established technology. If the Supercharger is located in front of the throttle body (blow through) as in the normally the case of a Centrifugal Compressor , and the throttle body closes at high rpm, the compressed air has no place to go, The resulting back pressure can cause surging through the Compressor and could damage this type of Supercharger. Since the Centrifugal Supercharger is also driven by the engine crankshaft at a very high ratio and therefore compressor speeds, the higher the engine rpm the greater the air flow and more severe the problem. So with this design of Supercharger a BOV to release Boost pressure on throttle closure is employed, at idle the BOV has Calibrated springs to allow Compressor Bypass and recirculation.
Positive displacement superchargers (Roots & Twin Screw)* are always moving air when rotating and would create a boost element by their design and as such they are usually positioned downstream of the throttle body (suck through) and in almost all situations they have a Bypass valve. The Bypass valve negates most of the work of compressing/moving the air (Pressure, Heat which the engine has to provide work to produce) during idling or part throttle non boost operation. The bypass valve is held “open” by engine vacuum, therefore with a Bypass valve the Supercharger’s parasitic losses are also substantially reduced under normal vacuum (10″-22″Hg). This allows the compressed air from the Supercharger outlet to re-circulate or bypass to the inlet thereby lowering the air temperature and power required to drive the Supercharger. When the throttle is opened and the Supercharger is called on to produce boost, the bypass valve closes off the recirculating air thereby directing all the boosted air into the engine. If the throttle body is closed at high rpm, there is no surge problem as with a Centrifugal compressor (blow through throttle, Centrifugal compressors charge air reacts against the closed throttle plate) This deceleration won’t harm the Positive displacement Supercharger in any way albeit create large vacuum, However, when coupled to an under bonnet filter that sucks in hot air, the heat may be a problem for any Supercharger – Centrifugal, Roots or Twin Screw, Hot air supplied to the Supercharger is less dense losing potential power, it raises the chance of Detonation in the cylinders and adds to the heat that the Supercharger operates at causing potential clearance/tolerance issues. We’ve said it before, avoid exposed under bonnet filters in Supercharged and/or all engines.*Please note that Roots type Superchargers are not imparting internal compression to the air charge, they are air movers.
SUPERCHARGING PRINCIPLES
-
- An engine will move a fixed volume of air, at a given speed, assuming WOT operation. This volumetric flow rate, or course, is entirely dependent on the engine size, speed, and volumetric efficiency. Items such as tuned length intake and exhaust systems, ported cylinder heads, valves, modified camshaft profiles are all designed to improve volumetric efficiency, which increases power.
- The AIR MASS flow rate through the engine is a product of inlet air density and volumetric flow rate. So, this means that for any given engine, one very effective way to get more air through it is to increase the density of the air available at the inlet.
- With increased AIR MASS flow rate, we can now add the correct proportional amount of additional fuel and produce more power. The important distinction is that power strongly depends on air density, i.e. the amount of oxygen available in a given volume to combine with fuel.
The fundamental purpose of Supercharging is to increase the gas (air) density at the inlet to the engine. That is essentially it.
THERMODYNAMICS OF COMPRESSION
If you were to look at a Graph of the Compressor Isentropic efficiency process it would be noticed this compression occurs along a vertical line on a Graph, it is termed "isentropic", which means that it is "ideal" or perfect. In other words, it occurs with no losses, albeit the temperature of the air (gas) rises with compression. It is therefore noticed that even an isentropic or ideal compression process results in a temperature rise. Note that some refer to this as the "heat of compression". This behaviour follows the "ideal gas law" relationship, which relates the pressure [P] temperature [T] and volume [V] of a gas with a constant of proportionality, called the gas constant [R]*. There are many possible Compressor processes that can occur, but all result in a greater temperature rise than the "ideal" process as it is impossible to construct a machine to produce a completely lossless, or perfect compression, hence actual compression curve. Even "ideal" compression results in temperature rise, according to the ideal gas law. As the process becomes less ideal, depending upon the design ( i.e. less "efficient" compressors) the temperature rise becomes greater. All processes, however, result in the same pressure, but with the increase in Temperature comes a decrease in charge density.
THE IMPORTANCE OF COMPRESSOR EFFICIENCY
Perfection would be the (impossible to achieve) ideal compression process, we can now consider more realistic compression processes that involve actual compressors or superchargers to accomplish the compression work. In this sense, we consider the "efficiency" of the actual compression relative to the ideal process, and call this the "isentropic efficiency". Now, an isentropic or ideal process would also be 100% efficient. Actual compressors, operate at efficiencies below this level. Also, the further the process deviates from the ideal, the less efficient it becomes, and, the greater the temperature rise.
- A less efficient compressor produces less density rise, at the same pressure. This follows from the ideal gas law relationship: p=P/RT
- A less efficient compressor absorbs more Crankshaft drive power, at the same pressure and flow rate.
- Therefore, and given these two properties, the most efficient compressor will produce the greatest net-power gain potential on the engine.
THE ESSENTIALS
Compressor or Supercharger efficiency must be at the top of the list of tested parameters - the engineer must be able to test the efficiency of his compressor design in order to assess how well he achieved the design task. The Tuner/consumer, on the other hand, wants the greatest potential net-power gain for his expenditure. Thereby making comparisons for the tested efficiencies of various competing products and decide accordingly, selecting the most efficient product will deliver the greatest potential net-power gain. Other important performance parameters;
- Range - This refers to the stable or usable flow range, when operating at a constant speed. Typically, the lowest flow point of a Centrifugal compressor is the "surge" line, where operating instabilities occur. At the high-flow end is the "choke" region, where the compressor can no longer maintain pressure. At either of these extremes, efficiency drops-off rapidly and overall performance suffers.
- Pressure - Clearly an important property as the fundamental purpose of the compressor is to develop increased pressure. so testing for pressure performance would entail operating at various rotar/impeller speeds from minimum to the safe maximum, and measuring pressure performance at the corresponding efficiency levels. It should be noted that some compressors by design cannot develop high pressure while also maintaining good efficiency; the temperature rise combined with the increased drive power, in fact, may be so excessive that a net loss in engine power may result.
- The Pressure versus Flow behaviour - Relevant to more advanced studies, this refers to the shape of the individual pressure vs. flow curves. In many cases, a flat performance curve may be preferred as this offers improved flexibility for matching the compressor to various engines.
- Efficiency performance is entirely dependent on the design of the compressor - poorly designed compressors perform poorly, whether operating continuous and stabilized, or dynamic.
- A more efficient compressor, will always deliver cooler charge air and consume less engine power than an inefficient compressor, at the same (flow and pressure) operating point. This fact holds whether the compressor is running continuously at the operating point, or rapidly transitions through it.
COMPRESSOR MAPS
The compressor map represents the most significant performance results from testing in a very clear and useful manner. Multi point data is displayed and includes the pressure versus flow curves, each obtained at a fixed operating speed, and the all-important Iso-efficiency contours, which are often referred to as efficiency islands. The term "map" is quite appropriate as, usually, the useful operating range of the compressor is displayed in its entirety and full knowledge of compressor performance can be obtained at any specific operating point. With a compressor map, one can also over-plot an engine air demand curve and determine whether the compressor is suitably matched to the engine.
EFFICIENCY ISLANDS
For each data point logged, there is also a corresponding efficiency. The efficiency islands are lines of constant efficiency, which are over-plotted on the pressure vs. flow map. They are obtained through a graphical construction process from the efficiency vs. flow curves.
SURGE LINE CENTRIFUGAL COMPRESSORS
Surge is the left hand boundary of the compressor map. Operation to the left of this line represents a region of flow instability. This region is characterized by mild flutter to wildly fluctuating boost and “barking” from the compressor. Surge is most commonly experienced when one of two situations exist. The first and most damaging is Surge under load. Surge is also commonly experienced when the throttle is quickly closed after boosting, resulting in rapid airflow stall/reversals and pressure fluctuations.
CHOKE LINE CENTRIFUGAL COMPRESSORS
The Choke Line is the right hand boundary of the compressor map. The choke line is typically defined by the point where the efficiency drops below 58%. Due to the rapid drop of compressor efficiency past this point, If your actual or predicted operation is beyond this limit, a larger compressor is necessary
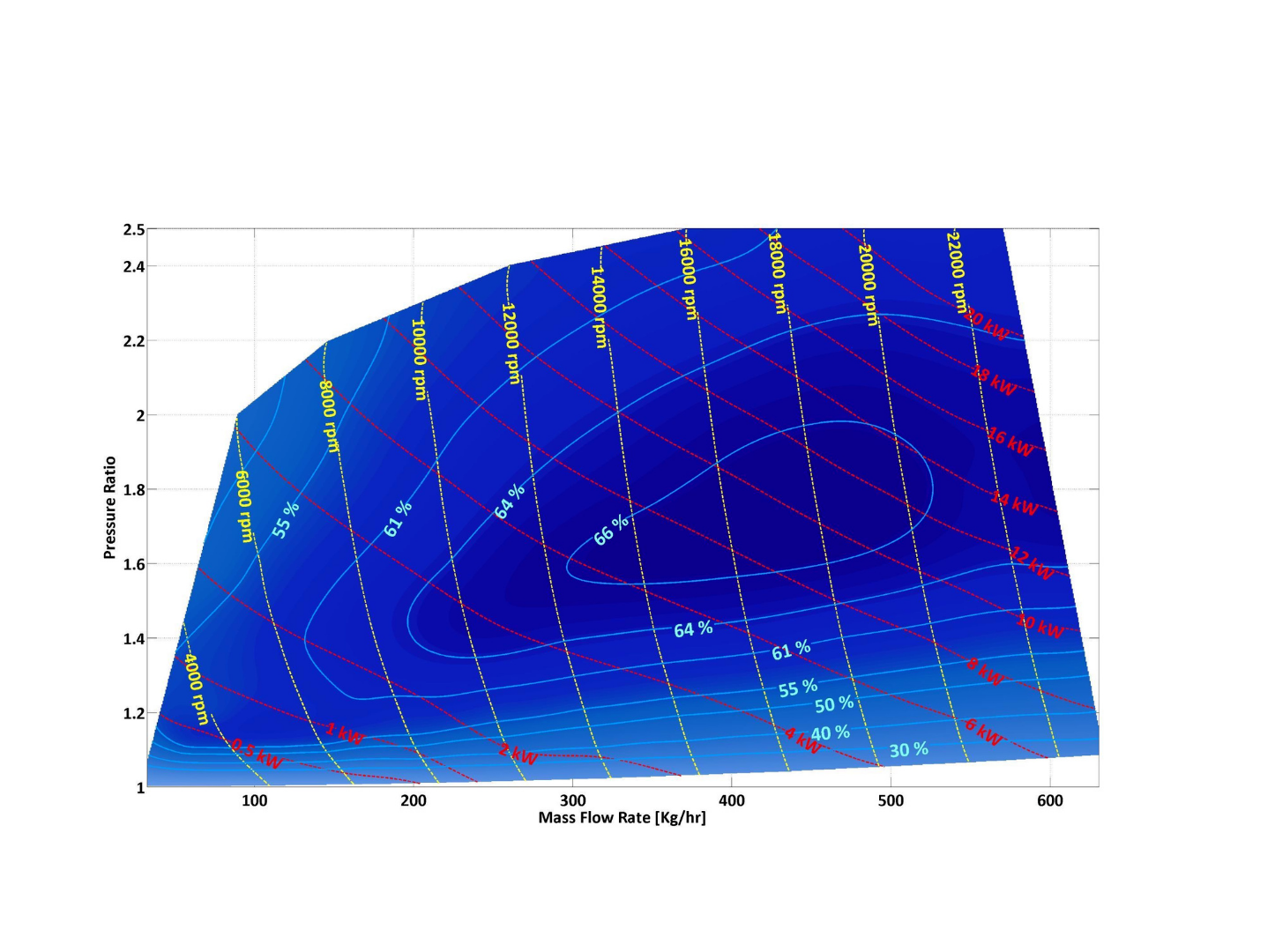
PRESSURE RATIO (PR)
The term Pressure Ratio is simply the absolute discharge pressure divided by the absolute inlet pressure. By plotting pressure rise as a ratio, one can quickly and conveniently determine the discharge pressure of the compressor, when the inlet pressure is known. PR is to be computed using the stagnation or "total" pressure measurements. The total measurements differ from static measurements in that the kinetic energy of the high velocity air is accounted for and incorporated. In essence, then, this computes the complete change in air energy state from the compressor inlet to the outlet. Gauge Pressure measures the pressure above atmospheric, so a gauge pressure reading at atmospheric conditions will read zero. Boost gauges measure the manifold pressure relative to atmospheric pressure, and thus are measuring Gauge Pressure.
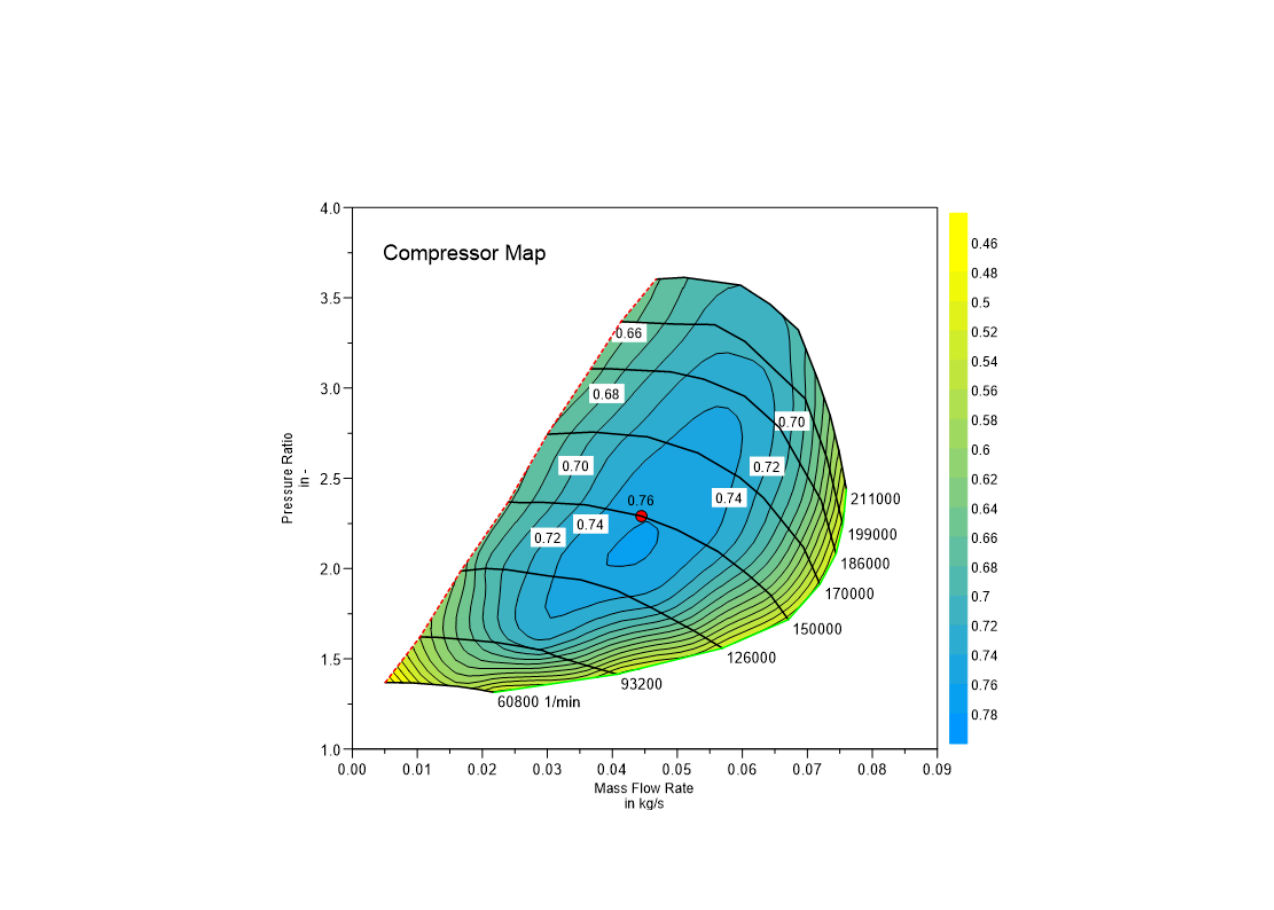
SPEED LINES - CENTRIFUGAL COMPRESSORS
These are lines of constant speed on the map, and indicate pressure rise vs. flow, at the indicated impeller speed. The lines are constructed by taking 5 to 7 data points, at various flows from surge to choke, and fitting a curve to these data to form the line. To develop a complete map, multiple speed lines are constructed to cover to the operating speed range of the supercharger. This also minimizes inaccuracies arising from interpolating the data. Thus, many stabilized operating points (perhaps 50 or more) must be logged in order to construct a complete map.
COMPRESSION RATIO WITH BOOST
It is important to understand engine knock, also known as Detonation. Detonation is a dangerous condition caused by uncontrolled spontaneous combustion of the air/fuel mixture. This abnormal combustion causes rapid spikes in cylinder pressure and temperatures at the wrong time which can result in engine damage very quickly.
Three primary factors that influence engine Detonation are:
- Detonation resistance characteristics (knock limit) of the engine: Since every engine is vastly different when it comes to Detonation resistance, there is no single answer to “how much.” Design features such as combustion chamber geometry, valves, spark plug location, bore size and compression ratio all affect the Detonation characteristics of an engine. But as a general rule the lower the Geometric compression ratio the higher the potential Boost pressure, it all relates to peak cylinder pressures and temperatures. For this reason forced induction engines are generally below 10:1 compression ratio with high boost engines lower than this, perhaps 7.5:1- 8.0:1. The combination of Geometric compression ratio and Boost pressure combine to give a higher effective compression ratio.
- Ambient air conditions: For the Supercharger application, both ambient air conditions and engine inlet conditions affect maximum boost. Hot air and high cylinder pressure increases the tendency of an engine to Detonate. If the Compressor inlet is being fed an air source that is well above the atmospheric ambient temperature then it is compounding the problem. When the compressor is providing boost to the engine, the manifold intake charge air temperature increases above the ambient it is sourced from due to the work imparted on the air through compression and thus potentially increasing the tendency to Detonate. Charge air cooling (e.g. an Intercooler) helps to address this issue by cooling the compressed air produced by the Supercharge reducing its temperature and aiding density recovery, but supplying air that is as cold as possible in the first place aids power and safety.
- Octane rating of the fuel being used: Octane is a measure of a fuel’s ability to resist Detonation. The Octane rating for pump fuel depends on supplier and country, in the UK 95Ron 97Ron or 99Ron and racing fuel is most likely well above 100. The higher the Octane rating of the fuel, the more resistant to Detonation. Which is important due to the fuel in most Petrol engines already being in the air charge as the Cylinder Compression stroke begins. As this mixture is Cylinder compressed into the Combustion chamber pressures and temperatures become very high, and in certain circumstances ignition can take place before the spark, or a valve or other hot spot could induce the same spontaneous pre spark ignition which can lead to extremely rapid collision of flame fronts, Detonation. Since Detonation can be extremely damaging to an engine due to the forces involved, it is important to use fuel of sufficient Octane for the application. Generally speaking, the more boost pressure, the higher the octane requirement and importance of fuel air ratio.
SUPERCHARGER SIZING
The efficiency of the Supercharger significantly reduces if an oversized Positive Displacement supercharger or centrifugal compressor has been fitted to an engine where by the Mass air delivery/engine requirements are not matched relevant to compressor map. The engine will not operate the oversized Supercharger fast enough for it to be in the best efficiency range relative to engine airflow requirements, as a consequence Horsepower and Torque will be down in the low and mid engine RPM ranges. The positive displacement type may behave much like a centrifugal supercharger wherein boost/air flow is totally dependent and proportional to engine RPM. Then a slow boost rise curve may then become an issue. With an oversized Centrifugal compressor the airflow requirements of the engine will be towards the left hand side of the compressor flow map, which leads to operating at lower efficiencies and more importantly very close to the Surge line which will give unstable airflow through the compressor in some circumstances and with throttle closure a probability of going into compressor surge.
Conversely to this having a Supercharger that is too small (Mass airflow Lbs/Min, Kg/Sec) in delivery placed onto an engine with specific airflow/boost needs will need to be rotated at speeds that can be beyond bearing and seal limits in Rpm terms. The unit may be of a design that as rotational speeds become excessive, it creates huge losses internally due to the airspeeds, Choke and the pressure ratio it is expected to deliver. It is most likely operating well outside of best efficiency zones and therefore it excessively heats the charge air delivery which reduces charge air density.
MASS FLOW RATE
- Mass Flow Rate is the mass of air flowing through a compressor (and engine!) over a given period of time and is commonly expressed as Lb/min (pounds per minute) Kg/Sec or M3/sec Mass flow can be physically measured, but in many cases it is sufficient to estimate the mass flow for choosing the correct Supercharger.
- Some Tuners use Volumetric Flow Rate (expressed in cubic feet per minute, CFM or ft3/min) instead of mass flow rate. Volumetric flow rate can be converted to mass flow by multiplying by the air density. Air density at sea level is 0.076lb/ft3 depending on the Temperature.
- As a general rule, Supercharged Petrol engines will generate 9.5-10.5 horsepower (as measured at the flywheel) for each Lb/min of airflow. So, an engine with a target peak horsepower of 400 Hp will require 36-44 Lb/min of airflow to achieve that target. This is a first approximation to aid Supercharger selection.
AIR/FUEL RATIO : Rich vs Lean
When discussing engine tuning the ‘Air/Fuel Ratio’ (AFR) is one of the main topics. Proper AFR calibration is critical to performance and durability of the engine and its components. The AFR defines the ratio of the amount of air consumed by the engine compared to the amount of fuel.
When utilising a Supercharger to increase the air density in an engine, a higher mass of fuel will be required to maintain the Stoichiometric mixture, and for most engines this is generally accepted as 14.68:1 Air Fuel ratio. With Supercharged engines however this ratio tends to be much Richer, the terms of Rich or Lean relate to the amount of Air to Fuel and have significant impressions on engine performance and reliability, so what mixture ratio is desirable?
A Liquid, such as petrol as it transforms from a liquid state to vapour absorbs heat, the amount of heat it absorbs is called Latent Heat of Vaporisation. So, with a Rich mixture this occurrence can be taken advantage of for cooling purposes within the Supercharged Cylinder, a ratio in the area of 11.7:1 lowers peak cylinder temperatures, slows the flame front, burns cooler, adds an element of safety against Detonation, preserving engine components and can possibly allow slightly more ignition advance. There is of course a limit to how Rich, overly Rich mixtures can lead to much higher emissions, Spark Plug fouling and potential for Cylinder Bore oil wash.
Conversely a Lean Air Fuel ratio will have the opposite effect, a Lean Fuel ratio will absorb less heat due to the lesser quantity of fuel and potentially promote Detonation. Lean Air Fuel ratios have faster flame fronts, higher burn temperatures that can significantly raise Cylinder and exhaust gas temperatures which in turn can lead to component failure.